- 1Division of Pediatric Neurosurgery, Pediatric Clinical Neuroscience Center, Seoul National University Children’s Hospital, Seoul, South Korea
- 2Department of Neurosurgery, Seoul National University Hospital, Seoul National University College of Medicine, Seoul, South Korea
- 3Department of Anatomy, Seoul National University College of Medicine, Seoul, South Korea
Purpose: Atypical teratoid/rhabdoid tumor (AT/RT) is arising typically in young children and is associated with a dismal prognosis which there is currently no curative chemotherapeutic regimen. Based on previous studies showing high histone deacetylase 1 (HDAC1) expression in AT/RT, the HDAC1 inhibitor CI-994 was used as a novel treatment strategy in this study. We assessed the anticancer effects of CI-994 and conventional drugs (etoposide, cisplatin or 4-HC) in AT/RT cells.
Methods: AT/RT patient-derived primary cultured cells and cell lines were prepared. HDAC1 was estimated by real-time quantitative polymerase chain reaction (RT-qPCR). The interaction of the drugs was analyzed using isobologram analysis. Cell viability, apoptosis, HDAC enzyme activity and western blot assays were carried out.
Results: HDAC1 was overexpressed in AT/RT compared to medulloblastoma. The combination index (CI) of CI-994 with etoposide revealed a synergistic effect in all AT/RT cells, but no synergistic effect was observed between CI-994 and cisplatin or 4-HC. CI-994 effectively reduced not only Class I HDAC gene expression but also HDAC enzyme activity. The combination treatment of CI-994 with etoposide significantly increased apoptosis compared to the single treatment. The enhanced effect of apoptosis by this combination treatment is related to a signaling pathway which decreases topoisomerase (Topo) II and increases histone H3 acetylation (Ac-H3).
Conclusion: We demonstrate that the combination treatment of CI-994 with etoposide exerts a synergistic anticancer effect against AT/RT by significantly inducing apoptosis through Topo II and Ac-H3 regulation.
Clinical Relevance: This combination treatment might be considered a viable therapeutic strategy for AT/RT patients.
Introduction
Atypical teratoid/rhabdoid tumor (AT/RT) is one of the most malignant pediatric brain tumors that typically arises in infants younger than 3 years old (1). Maximal safe resection followed by multimodal therapy is recommended as the standard treatment. However, the prognosis of patients with AT/RT is still poor (2, 3). The difficulty of gross total resection, incomplete efficacy of intensive chemotherapy and limitation of radiotherapy for young patients highlight the urgency of developing novel therapeutic strategies (3).
AT/RT is characterized by biallelic loss-of-function alterations in SMARCB1, which encodes the hSNF5/BAF47/INI1 subunit of the SWI/SNF chromatin remodeling complex (3, 4). Previous in-depth molecular studies explained the observed clinical heterogeneity but relatively unaltered genome of AT/RT by noting substantial heterogeneity in epigenetic profiles (2, 3). The Toronto group (5) and German group (6) recently classified AT/RT into 3 molecular subgroups based on its epigenetic profiles from two different perspectives. Studies targeting the mechanisms of epigenetic regulation in AT/RT treatment have been extensively conducted, leading to successful use of histone deacetylase (HDAC) inhibitors such as trichostatin A, SAHA, and SNDX-275 (7). It also suggested that a specific class of HDAC inhibitors may be more effective for certain molecular classes of AT/RT (5).
HDAC regulates the expression of genes and proteins involved in both cancer initiation and progression (8), and high expression levels of several HDACs are associated with poor prognosis of cancer patients (9). Additionally, HDACs have been found to regulate cancer cell functions, including DNA damage, cell death and differentiation (10). Therefore, the anticancer effects of HDAC inhibitors have been evaluated in various cancers (11).
HDAC1, which is a Class I HDAC, has been reported to play important roles in epigenetic regulation for tumor progression and is significantly overexpressed in many cancers (10, 12), including AT/RT (13, 14). Importantly, HDAC1 is highly expressed in AT/RT tissues compared to normal cerebellum and CNS non-cerebellum (15). As a drug that can inhibit HDAC1, CI-994 (Tacedinaline, N-acetyldinaline) is an oral compound that is also a selective Class I HDAC inhibitor (16). CI-994 has been verified to exhibit significant anticancer activity against a broad spectrum of human cancers in vitro (17) and in vivo (16).
Many preclinical and clinical studies have examined rational combinations of HDAC inhibitors with many current therapies for the treatment of hematological and solid tumor malignancies (18). Notably, CI-994 was investigated in combination with other anticancer drugs in phase I/II clinical trials for solid tumors (19). Therefore, the potential benefits that CI-994 might confer in the treatment of AT/RT led us to investigate the combination treatment of CI-994 with conventional anticancer drugs.
In this study, we evaluated the combination treatments of CI-994 with three different conventional chemotherapeutic agents (etoposide, cisplatin, or ifosfamide) commonly used in two protocols for the treatment of AT/RT (2). As etoposide showed the most potent synergistic effect with CI-994, we investigated the potential signaling pathway affected by this combination that leads to its anticancer effects.
Materials and Methods
Patients and Samples
Brain tumor tissues (Table 1) were collected from patients diagnosed with AT/RT (N=13) and MBL (N=13) who underwent initial surgery at the Seoul National University Children’s Hospital. The Institutional Review Board (IRB) of the Seoul National University Hospital (SNUH) approved the study protocol (IRB approval No. 1707-095-878). The pathological diagnosis of AT/RT was made histologically and confirmed by the lack of INI-1/SMARCB1 protein expression. The AT/RT subgroup was determined by immunohistochemistry staining of tissues (6). The molecular groups of medulloblastoma were analyzed by NanoString nCounter (20).
Real-Time Quantitative Polymerase Chain Reaction (RT-qPCR)
Total RNA was isolated using the miRNeasy Mini Kit (Qiagen, Hilden, Germany), and cDNA was synthesized using the EcoDry Premix kit (Clontech, Mountain View, CA) (21). RT-qPCR assay was performed by a TaqMan assay on an ABI 7500 system (Applied Biosystems, Foster City, CA) using TaqMan probes for HDAC1, HDAC2, HDAC3, HDAC8, and GAPDH. The relative expression levels in each sample were calculated and quantified by using the 2-ΔΔCT method. The value of each control sample was set to one and was used to calculate the fold change in target gene expression. GAPDH was utilized to normalize the gene expression results.
Immunohistochemistry (IHC)
The expression of HDAC1 protein within tissues were verified by IHC as previously described (6). A total of 8 cases of tissue (4 cases in medulloblastoma and 4 cases in AT/RT) used to verify HDAC1 protein expression. Of these, 3 cases of each group were newly obtained, and 1 case of each group was included in the previous RT-qPCR analysis. Briefly, the sections were incubated with primary antibodies, HDAC1 (1:1000, Abcam, Cambridge, MA), for 32 min at 37°C, and a secondary antibody for 20 min at 37°C. The stained sections were detected using the Ventana ChromoMap Kit (Ventana Medical Systems) and discovered using XT automated IHC strainer (Ventana Medical Systems, Oro Valley, AZ).
Cell Culture
AT/RT primary cells were cultured as previously described (21). The cell lines of AT/RT (BT12 and BT 16) and MBL (UW228 and MED8A) were provided from Dr. Peter Houghton (Nationwide Children’s Hospital) and Dr. Young Shin Ra (Asan Medical Center, Seoul, Korea), respectively. The human neural stem cell HB1.F3 was used as a normal control. All cells were cultured in Dulbecco’s modified Eagle’s medium (DMEM; Welgene, Seoul, Korea) containing 10% fetal bovine serum and 1% antibiotic-antimycotics and incubated at 37°C in a 5% CO2 atmosphere.
Drugs
CI-994, etoposide, and cisplatin were purchased from Selleckchem (Houston, TX). We used the activated form of ifosfamide, 4-hydroperoxycyclophosphamide (4-HC), from Cayman (Ann Arbor, MI). The drugs were dissolved in dimethyl sulfoxide (DMSO) to generate 10 mM stock solutions and diluted to the indicated concentrations with culture medium before the experiments.
Cell Viability Assays
The median inhibitory concentration (IC50) was determined in AT/RT cells. The cells (4 × 103) were cultured in 96-well plates and exposed to various concentrations of the drugs (0-100 µM). Cells treated with 0.1% DMSO were used as a control. Cell viability was measured using the EZ-cytox kit (Daeil Lab Service, Seoul, Korea) after drug treatment for 72 h. The percentage of cell viability of the treated cells was measured relative to that of the control cells. Cell growth curves were drawn, and the IC50 was calculated by nonlinear regression analysis using Prism software (La Jolla, CA).
Isobologram Analysis
To evaluate the dose-responses of the CI-994-based combination treatments, an isobologram was drawn for each drug combination based on 5 constant ratios: 0.25× IC50, 0.5× IC50, IC50, 2× IC50, and 4× IC50 (22). The synergy, additivity or antagonism was calculated on the basis of the multiple drug effect equation and quantified by the combination index (CI) and fraction affected (Fa) according to the Chou-Talalay algorithm utilizing CompuSyn software (Paramus, NJ, www.combosyn.com) (22, 23). The CI values indicate synergistic (CI < 1), additive (CI = 1) or antagonistic effects (CI > 1). The Fa levels of 50% (Fa = 0.5), 70% (Fa = 0.7), and 80% (Fa = 0.8) inhibition were created to study the dose-dependent interaction of the drug combinations. Fa < 0.5 was regarded as irrelevant because a large fraction of the cell population showed proliferation and reduced growth inhibition.
HDAC Enzyme Activity Analysis
HDAC enzyme activity was assessed by an HDAC enzyme activity kit (Biovision, Mountain View, CA) (23). After 72 h of drug treatment, proteins (50 μg) extracted from the cells was mixed with the assay substrate and incubated at 37°C for 1 h. The reaction was stopped by adding 10 μl of lysine developer and incubated for an additional 30 min at 37°C. Test samples were measured by a fluorimeter (Molecular Devices, Sunnyvale, CA) at 405 nm.
Apoptosis Analysis
Apoptosis was evaluated by the Annexin V-Fluorescein isothiocyanate (FITC)/propidium iodide (PI) binding assay kit (BD Biosciences, Franklin Lakes, NJ) according to the manufacturer’s instructions. After drug treatment for 48 h, the cells (1 × 106 cells/ml) were harvested, stained with Annexin V and PI in the dark for 15 min, subjected to FACSCanto (BD), and analyzed by FlowJo software.
Western Blot
Total proteins were extracted using radioimmunoprecipitation (RIPA) lysis buffer. Western blotting was performed using the iBlot system (Invitrogen) as previously described (23). The following primary antibodies were used: topoisomerase II (Topo II, 1:5000, Abcam), acetylated histone H3 (Ac-H3, 1:2000, Abcam), γ-H2AX (1:5000, Abcam), cleaved Parp (1:1000, Cell Signaling Technology, Danvers, MA), active Caspase-3 (cleaved Caspase-3, 1:100, Millipore, MA), Survivin (1:5000, Abcam), NF-κB (1:500, Abcam), C-Myc (1:10000, Abcam) and β-actin (1:5000, Sigma-Aldrich, St. Louis, MO). The blots were visualized by enhanced chemiluminescence (ECL, Invitrogen) with X-ray film. The band intensities were quantified using ImageJ software and normalized to β-actin.
Statistical Analysis
All the values were calculated as the mean ± SD or expressed as the percentage ± SD of the controls. Multiple group comparisons were performed by 1-way ANOVA. Differences between 2 groups were determined using a 2-tailed Student’s t-test and Mann-Whitney test. GraphPad Prism v7.0 software was used for all the statistical analyses. All the analyses were repeated at least three times, and differences were considered statistically significant at p < 0.05.
Results
Overexpression of HDAC1 in AT/RT
We first evaluated the mRNA expression levels of HDAC1 in AT/RT tissues and cells. Compared with MBL tissues, AT/RT tissues exhibited increased HDAC1 mRNA expression (2.19-fold, p < 0.05, Figure 1A) and protein expression (Figures 1C, D). In addition, we confirmed that there was no significant change in HDAC1 mRNA expression depending on the MYC subgroup (p= NS, Supplementary Figure S1). HDAC1 was more highly expressed in all AT/RT cells than in MBL cells (6-fold p < 0.05, Figure 1B).
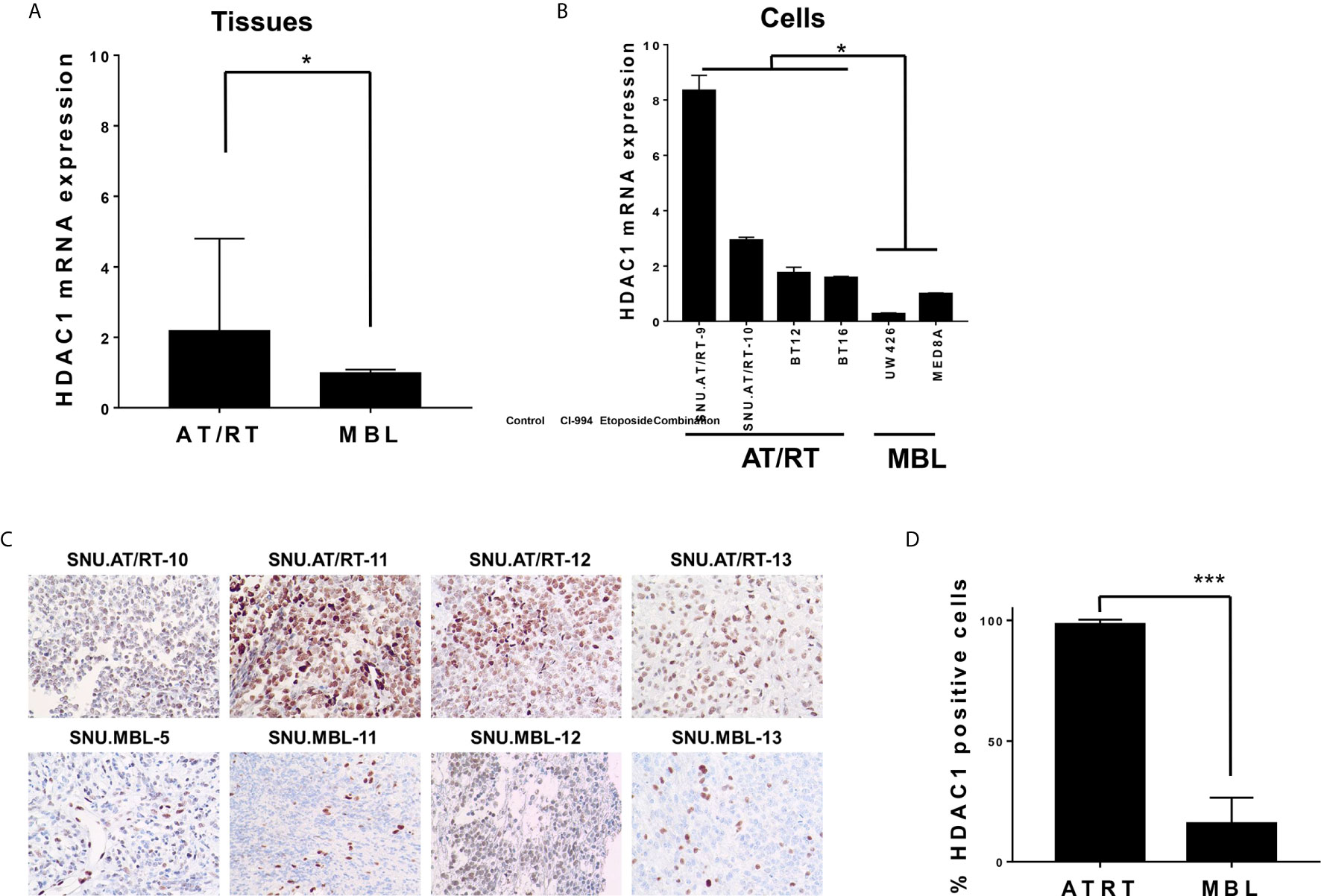
Figure 1 HDAC1 expression in AT/RT samples compared to MBL samples. (A) The quantitative polymerase chain reaction (qPCR) showed that HDAC1 mRNA expression in AT/RT tissues was 2.19 (p = 0.027) folds higher than in MB tissues. (B) HDAC1 mRNA level was tested in 3 AT/RT primary cultured cells(SNU.AT/RT-5, SNU.AT/RT-9, SNU.AT/RT-10) and each established AT/RT (BT12, BT16) and MB (UW426, MED8A) cell lines. HDAC1 is significantly overexpressed in AT/RT samples compared to the lowest expression level in UW426, one of MB cell lines. (C) IHC results show significantly higher HDAC1 protein expression in AT/RT compared to medulloblastoma. (D) The graph shows the percentage of HDAC1 positive cells in IHC (p < 0.0001). *p < 0.05, ***p < 0.0001.
Determination of IC50 Values
The prerequisite for confirming a synergistic effect is to determine the potency of each drug and the slopes of their concentration response curves. Therefore, we investigated the IC50 values of each drug in primary cultured AT/RT cells (SNU.AT/RT-9 and SNU.AT/RT-10) and AT/RT cell lines (BT12 and BT16). Increasing concentrations of each drug significantly reduced the viability of all AT/RT cells in a dose-dependent manner (Figure 2). The IC50 values ranged from 7.5 ± 0.2 to 65.0 ± 22.0 µM for CI-994, 4.9 ± 2.4 to 13.4 ± 4.3 µM for etoposide, 1.0 ± 0.05 to 56.1 ± 7.5 µM for cisplatin, and 5.3 ± 0.2 to 57.4 ± 5.0 µM for 4-HC in AT/RT cells (Table 2). The IC50 values of HB.F3 cells were 48.1 ± 26.6 µM for CI-994, 16.3 ± 5.2 µM for etoposide, 62.8 ± 3.8 µM for cisplatin, and 25.7 ± 11.1 µM for 4-HC. Compared to AT/RT cells, HB.F3 cells were more resistant to etoposide and cisplatin.
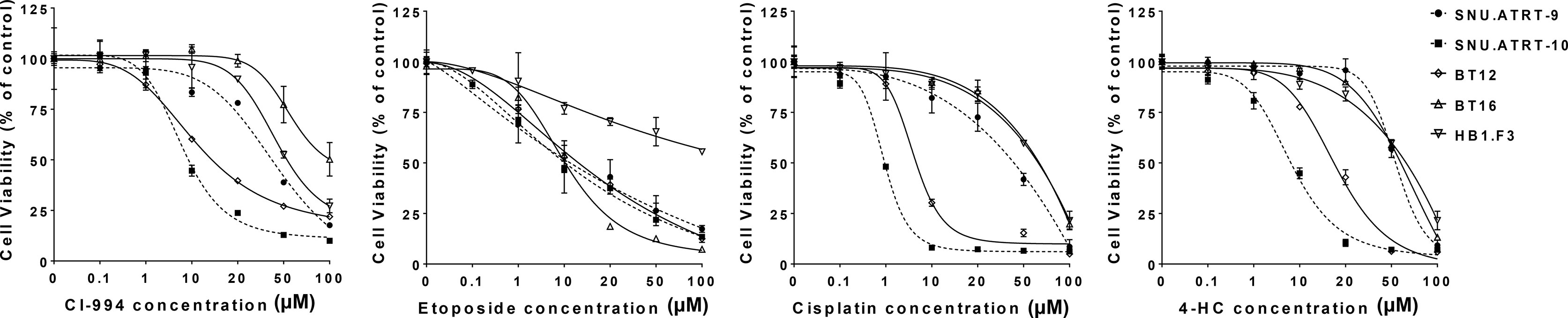
Figure 2 Single treatment in AT/RT cells. The viability of AT/RT cells against selected drugs (CI-994, etoposide, cisplatin, or 4-HC) was assessed by estimating their IC50.
Synergistic Effect of the Combination Treatment of CI-994 With Etoposide Against AT/RT
To determine the drug interaction of CI-994 with conventional chemotherapeutic agents, we calculated the fraction affected (Fa) and combination index (CI) values. The effect of the combination treatment of CI-994 with etoposide in all AT/RT cells was interpreted as synergistic: 0.3-0.54 in SNU.AT/RT-9 cells, 0.09-0.13 in SNU.AT/RT-10 cells, 0.5-0.8 in BT12 cells and 0.32-0.49 in BT16 cells (Figure 3A and Supplementary Table S1). The combination treatment of CI-994 with cisplatin or 4-HC did not exert synergistic effects in some cells (Figures 3B, C). In particular, antagonism was observed in SNU.AT/RT-10 cells with the combination treatment of CI-994 with cisplatin (Supplementary Table S2) and in SNU.AT/RT-10 cells and BT12 cells with the combination treatment of CI-994 with 4-HC (Supplementary Table S3). Therefore, etoposide was chosen as the drug to be combined with CI-994.
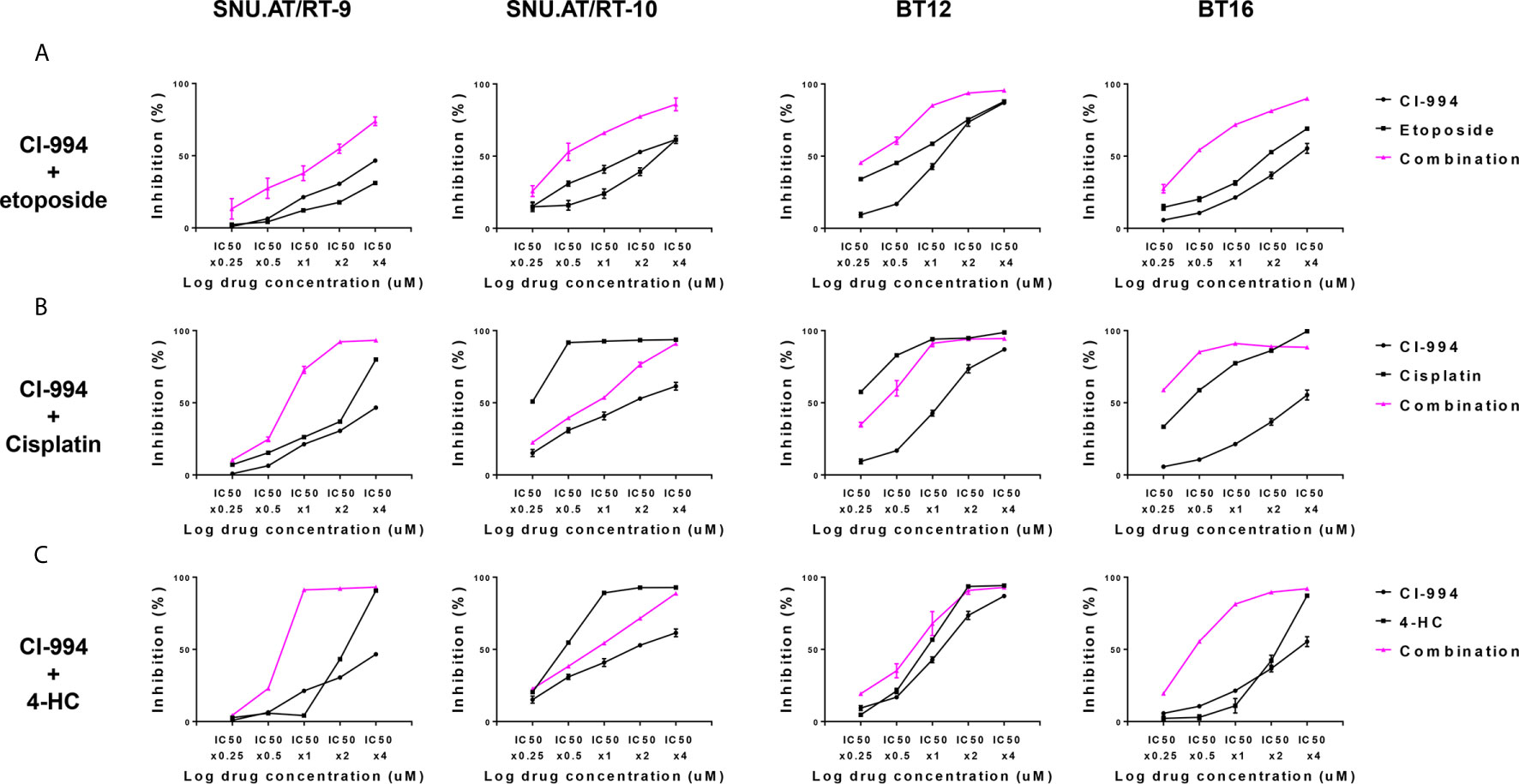
Figure 3 Comparative evaluation of the combination effect of CI-994 with conventional anticancer drugs. Curve shift analysis of CI-994 with conventional drugs (etoposide, cisplatin and 4-HC) in AT/RT cells shows that the degree of left shift represented the amount of synergism associated with the indicated drug combination. Single treatment: Black lines, Combination treatment: Pink lines. (A) In response to the combination treatment of CI-994 with etoposide, synergistic effects were observed and inhibited the growth potential of all AT/RT cells in a dose-dependent manner. (B, C) The combination treatment of CI-994 with cisplatin or 4 HC exerted cell type-specific effects. Synergistic effects were observed in the cells treated with the combination of CI-994 with cisplatin only for SNU.AT/RT-9 cells and in cells treated with the combination of CI-994 with 4-HC for SNU.AT/RT-9 cells and BT-16 cells.
At the optimal concentration of the combination treatment of CI-994 with etoposide, dose-response plots and Fa-CI were generated to the confirm drug interaction. The combination dose-response curves of all the AT/RT cells treated with CI-994 and etoposide shifted to the left, which indicated synergism by lowering the IC50 equivalent (Figure 4A). The Fa-CI plot showed all points to be under the horizontal line, which equaled 1 of the CI value at all concentrations. In the case of BT16 cells, all the points were closer to the bottom line than the points of the other AT/RT cells. The data points below the line of additivity indicate synergism (Figure 4B). The viability of cells exposed to the combination treatment of CI-994 with etoposide was significantly reduced by approximately 1.95- to 4.8-fold compared to that of cells exposed to the single treatment in all AT/RT cells (p < 0.0001, Figure 4C).
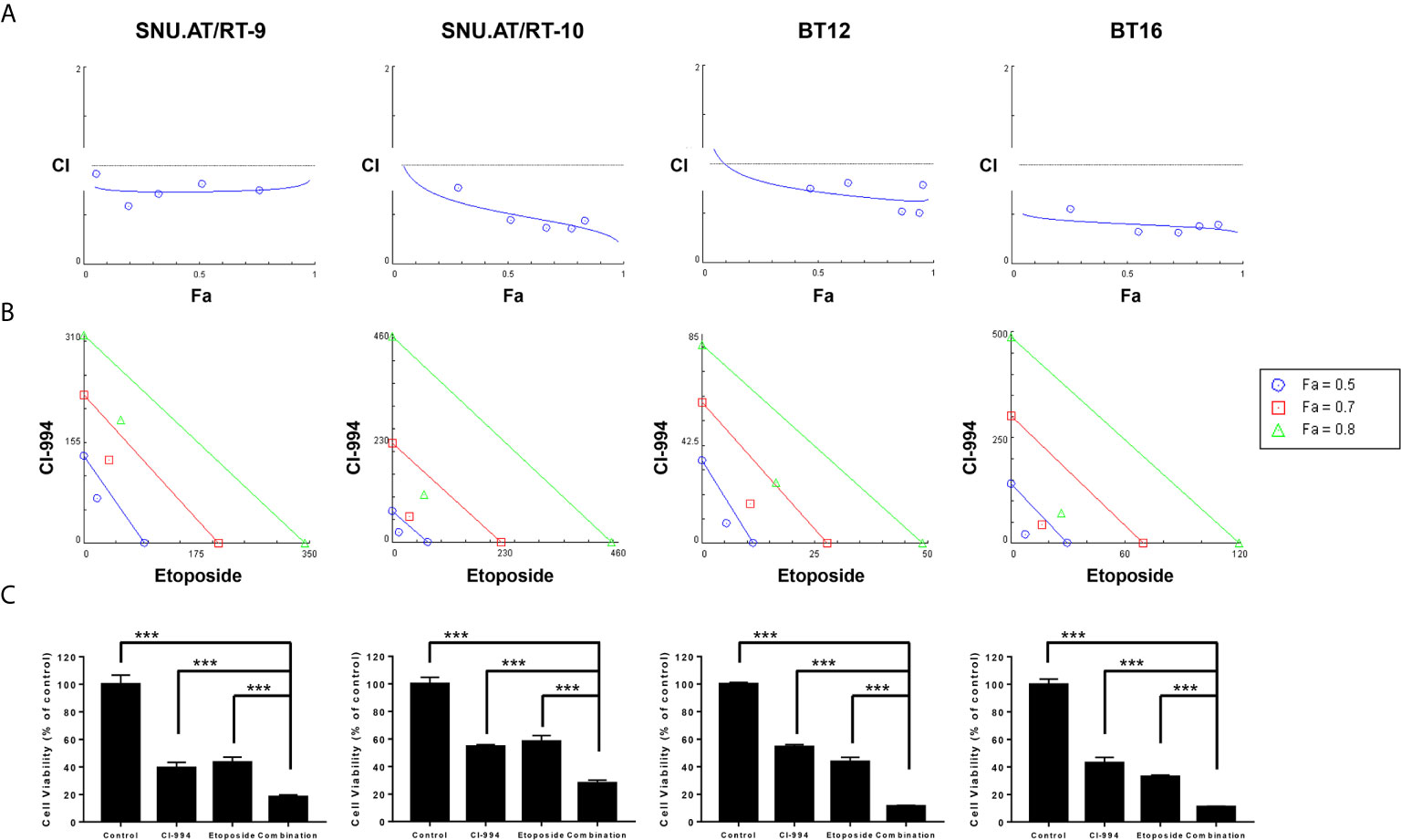
Figure 4 Synergistic effect of the combination treatment of CI-994 with etoposide in atypical teratoid/rhabdoid tumor (AT/RT) cells. Fraction affected vs. combination index (Fa-CI) plot was calculated for the combination of CI-994 with etoposide using an isobologram. (A) Dose-response curve shows that if the combination points (blue lines) lie below the gray line, there is a synergistic effect. Low CI values with increased Fa values suggest better compatibility and high synergism between CI-994 and etoposide. Graphs show a synergistic effect in all AT/RT cells. (B) The blue, red and green lines represent the theoretical additive line and the 0.5, 0.7 and 0.8 values, respectively. The synergy between CI-994 and etoposide increases as the calculated values approach the origin. In all AT/RT cells, synergism was observed at concentrations with Fa 0.5, Fa 0.7 and Fa 0.8 points. (C) Combination treatment of CI-994 with etoposide was significantly more effective than single treatment in all AT/RT cells. ***p < 0.0001.
Inhibition of HDAC1 mRNA Expression by CI-994
To confirm whether CI-994 effectively inhibits the mRNA expression of Class I HDAC, we performed RT-qPCR. As expected, HDAC1 expression was significantly decreased by CI-994 treatment alone and by the combination treatment compared to the control treatment in all AT/RT cells (Figure 5A and Supplementary Table S4). Etoposide single treatment did not affect HDAC1 mRNA expression. We also examined the mRNA expression of HDAC2, HDAC3 and HDAC8, which was significantly decreased by the CI-994 single treatment and combination treatment. In some AT/RT cells, HDAC2, HDAC3 or HDAC8 expression was reduced by etoposide single treatment.
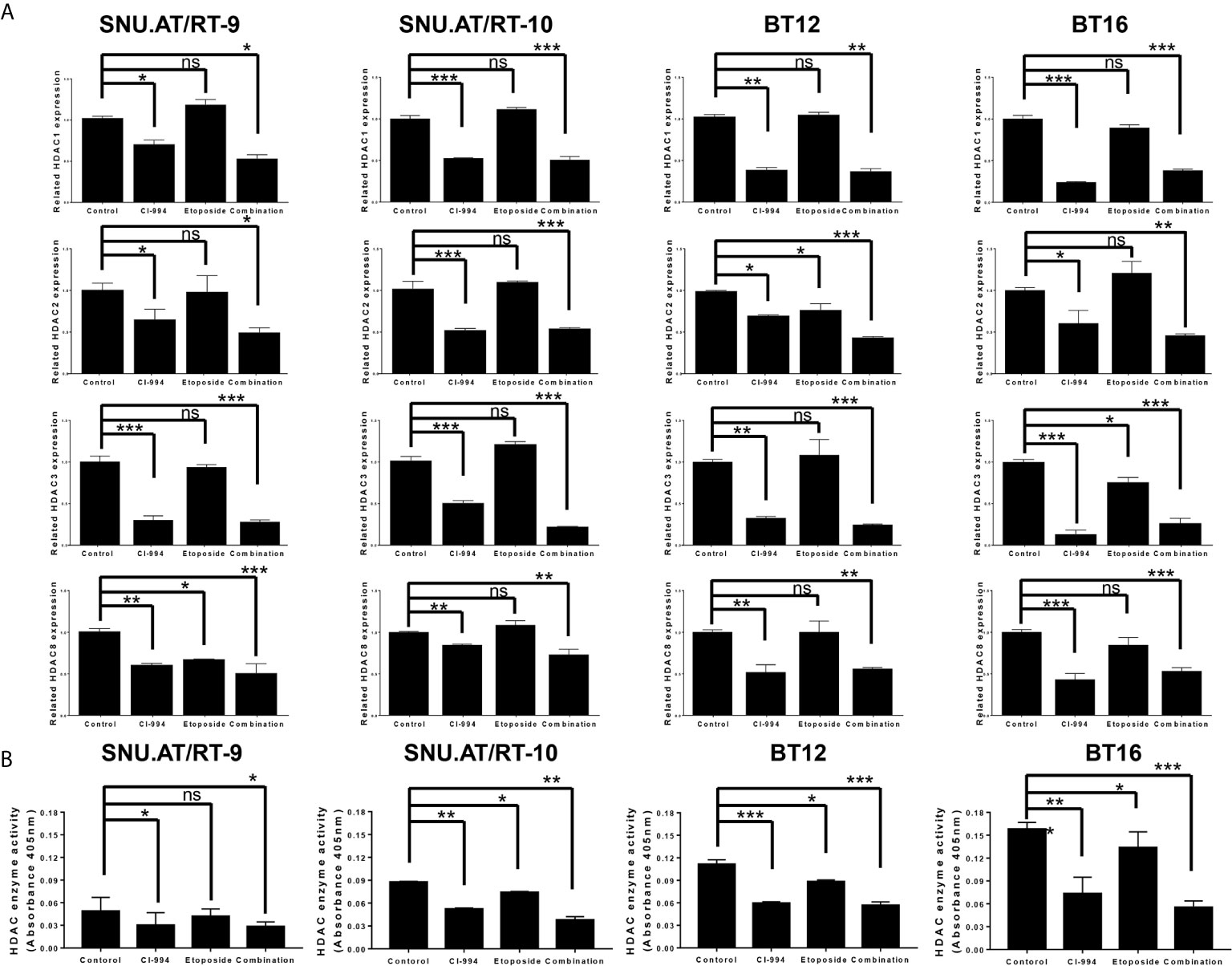
Figure 5 Class I histone deacetylase (HDAC) mRNA expression and HDAC enzyme activity following combination treatment of CI-994 with etoposide. (A) Class I HDAC expression was measured by RT-qPCR in AT/RT cells. Class I HDAC mRNA expression was significantly reduced by CI-994 treatment in all AT/RT cells. HDAC1 mRNA expression was not affected by etoposide single treatment in all AT/RT cells, but HDAC 2, 3, and 8 expression tended to decrease in some cells. (B) HDAC enzyme activities were significantly reduced by the CI-994 treatment and combination treatment. Even etoposide single treatment led to a slight decrease in SNU.AT/RT-10 cells, BT12 cells, and BT16 cells, but not in SNU.AT/RT-9. *p < 0.05, **p < 0.001, ***p < 0.0001, ns, not significant.
Decreased Activity of HDAC Following Combination Treatment of CI-994 With Etoposide
The inhibitory effect of the combination treatment of CI-994 with etoposide on HDAC activity was evaluated. Compared to the control treatment, the CI-994 single treatment and combination treatment effectively decreased the enzyme activities in all AT/RT cells (Figure 5B and Supplementary Table S5). Interestingly, the etoposide single treatment slightly suppressed HDAC enzyme activity in SNU.AT/RT-10 cells, BT12 cells, and BT16 cells, but not in SNU.AT/RT-9 cells.
Enhanced Apoptosis Following Combination Treatment With CI-994 and Etoposide
To confirm whether the synergistic anticancer effect of the combination treatment of CI-994 with etoposide is associated with apoptosis, we analyzed the proportion of apoptotic cells by Annexin V-FITC/PI binding assay. The percentage of apoptotic cells was significantly increased in all AT/RT cells treated with CI-994, etoposide and the combination. Importantly, the combination treatment induced more early apoptosis than CI-994 or etoposide single treatment in all AT/RT cells (Figures 6A, B and Supplementary Table S6).
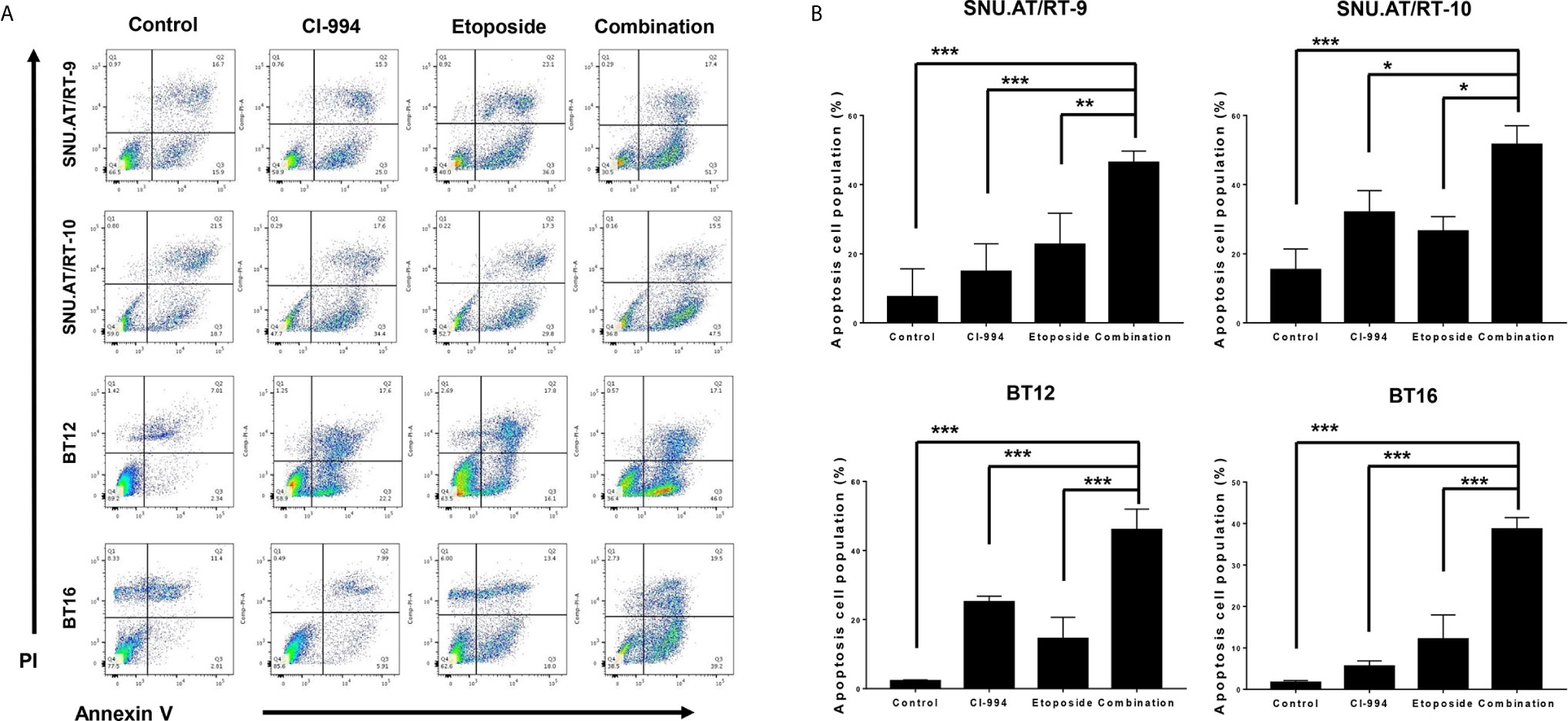
Figure 6 Effect of the combination treatment of CI-994 with etoposide on apoptosis in AT/RT cells. (A) Apoptosis in AT/RT cells was analyzed by Annexin V-FITC/propidium iodide assay and flow cytometry. (B) Percentages of apoptotic cells are presented on graphs. Combination of CI-994 with etoposide more considerably induced apoptosis in all AT/RT cells than the single treatment. *p < 0.05, **p < 0.001, ***p < 0.0001.
Decreased Topoisomerase II Expression and Increased Histone H3 Acetylation Following Combination Treatment With CI-994 and Etoposide
To investigate the molecular mechanisms associated with the synergistic anticancer effect of the combination treatment of CI-994 with etoposide on AT/RT cells, we explored the signaling pathways associated with DNA damage induced by Topo II and H3 acetylation (Ac-H3). In the majority of AT/RT cells, compared with the control and single treatments, the combination treatment led to decreased protein expression of Topo II and increased expression of Ac-H3, γ-H2AX, cleaved Parp, and cleaved Caspase-3 (Figure 7 and Supplementary Figure S2). Survivin was decreased in response to the single and combination treatment in most AT/RT cells; however, in BT16 cells, Survivin expression was increased in response to the etoposide single treatment. Since CI-994 increased Ac-H3 expression, Ac-H3 expression may induce the expression of the DNA damage-related protein γ-H2AX and initiation of early apoptosis, which increases the levels of cleaved Parp and Caspase-3. We also confirmed changes in the expression of NF-κB and C-Myc after drug treatment. The combination treatment of CI-994 and etoposide did not show any difference in the expression of NF-κB (Supplementary Figure S3A) but C-Myc (Supplementary Figure S3B) was effectively decreased in all AT/RT cells. On the other hand, the etoposide single treatment did not affect Ac-H3 expression but increased γ-H2AX expression. These data suggested that the combination treatment enhanced DNA breakdown by decreasing Topo II expression and increasing Ac-H3 expression, which potentiated apoptosis (Figure 8).
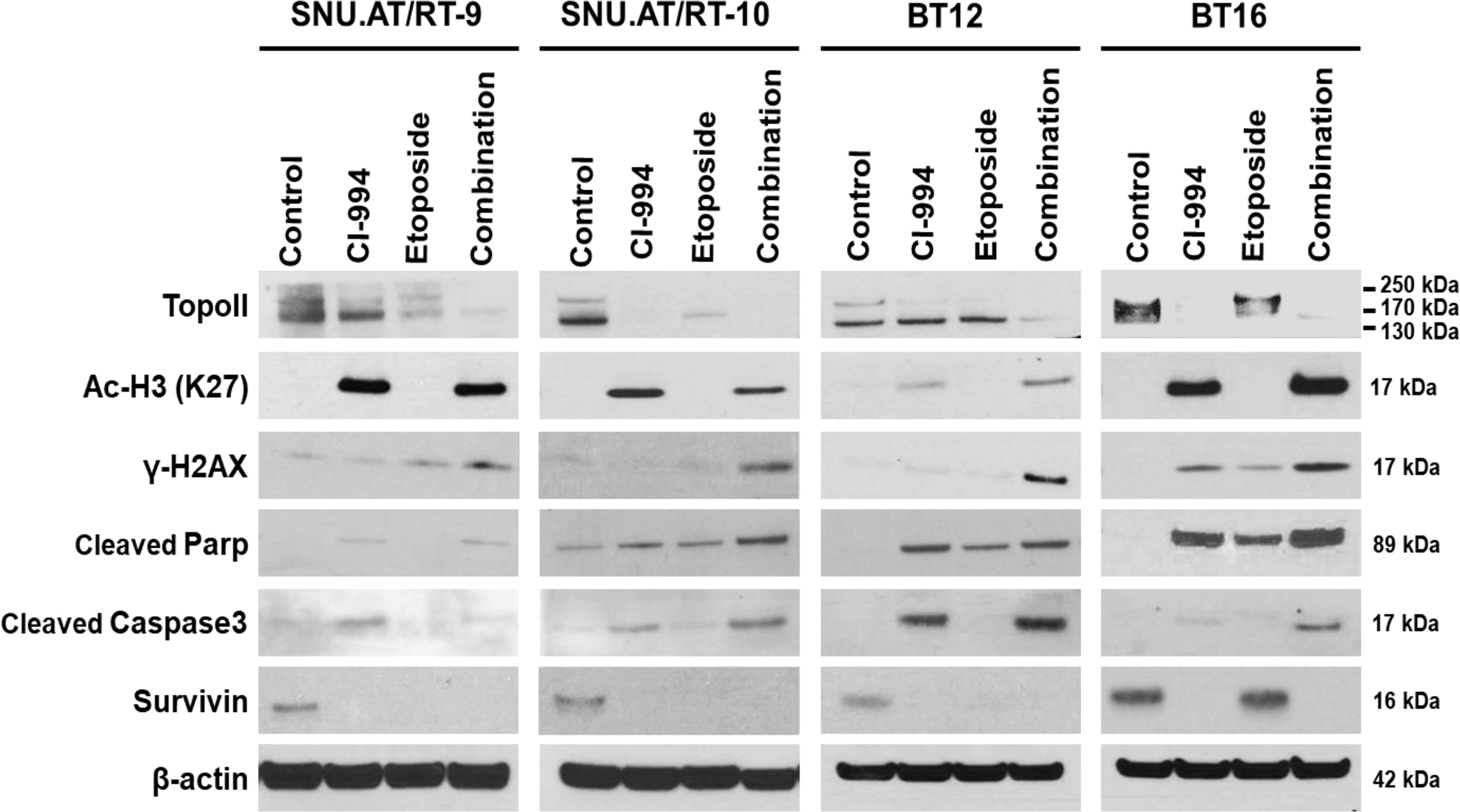
Figure 7 Protein expression involved in the synergistic effect of the combination treatment of CI-994 with etoposide. Western blot analysis shows the levels of topoisomerase (Topo) II, histone 3 acetylation (Ac-H3), γ-H2AX, cleaved Parp, cleaved Caspase-3 and Survivin in response to the combination treatment of CI-994 with etoposide.
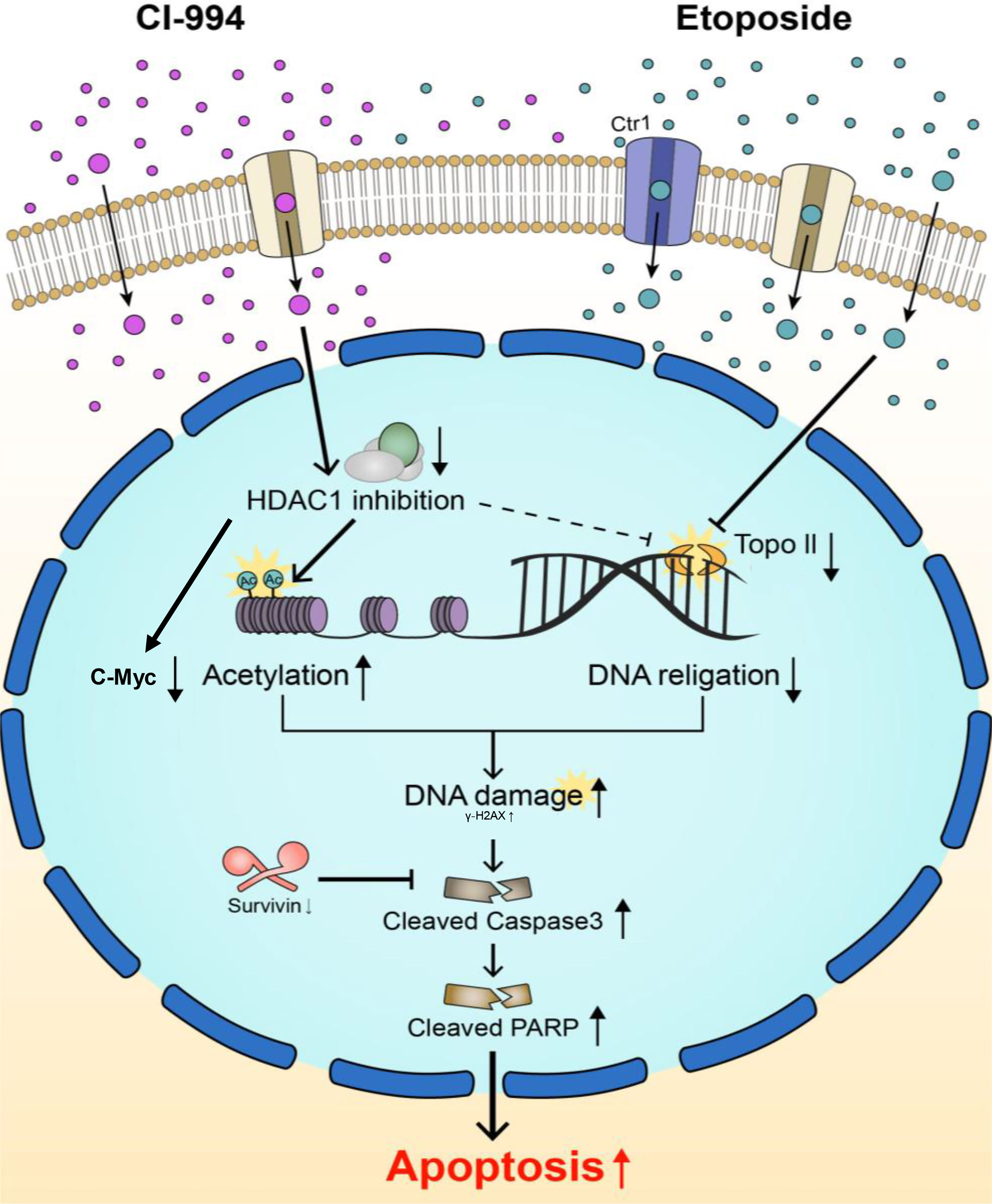
Figure 8 Schematic diagram of two main targets of the combination treatment. Topo II and HDAC1 are known to interact with each other and modify each other’s activity. CI-994 and etoposide can pass through cell membranes using transporters and/or passive diffusion. CI-994 enters the cell, inhibits HDAC1 enzyme activity and increases histone acetylation, therefore activating cleaved Parp. CI-994 also reduced C-Myc, which was more effective in combination treatment with etoposide. CI-994 can also increase γ–H2AX expression. Etoposide increases γ-H2AX expression, which not only increases cleaved Parp levels but also decreases Survivin levels. The action of each of these drugs through the regulation of Topo II and Ac-H3 synergistically increases cleaved Parp levels and cleaved Caspase-3 levels, leading to the apoptosis of AT/RT cells.
Discussion
Our study demonstrated the synergistic anticancer effect of the combination treatment of CI-994 with etoposide on AT/RT. The underlying mechanism of action was thought to occur through enhanced apoptosis due to decreased expression of Topo II and increased expression of Ac-H3.
The importance of HDAC-targeted therapeutics in various tumors (8, 24) has led to the extended use of specific HDAC inhibitors in pediatric brain tumors, including AT/RT (14). Previous studies showing HDAC1 overexpression in AT/RT (13) and verification in our samples provided rationale for the use of HDAC1 inhibitors. Since there are no commercially available inhibitors that regulate only HDAC1, we used CI-994, which is a relatively selective HDAC1 inhibitor (25, 26). It would be difficult to target and selectively regulate only HDAC1 because HDAC1, 2, 3 and 8, which are Class I HDACs, interact with each other (27). This limitation highlights the importance of intensive research on the development of HDAC single isoform inhibitors. This is one of the limitations of our study and remains a challenge to be addressed.
The three molecular subgroups of AT/RT are well known, and this should be taken into account as different subgroups may induce different drug sensitivities (28). The subgroups of AT/RT cells we used in this study were different (SNU.AT/RT-9: TYR/MYC, SNU.AT/RT-10: TYR, BT12: TYR, BT16: controversial). It should be kept in mind that the sensitivity of the drug may vary depending on the subgroup.
The penetration of CI-994 through the blood-brain barrier (BBB) is low (permeability surface area products BBB: 12.7 ± 0.1 μL/min/g brain) (29). Therefore, delivery strategies via intratumoral (30), intracisternal (31) and intranasal (32) injections may be required to bypass the BBB.
Many preclinical studies have suggested that the combination treatment of HDAC inhibitors with conventional chemotherapeutics safely shows synergistic effects even at lower concentrations (18). Recent studies have suggested that the use of HDAC inhibitors may be beneficial for the treatment of children with AT/RT as part of multimodal therapies (14). Although CI-994 is a potential anticancer drug, it is associated with dose-dependent toxicity and side effects, including thrombocytopenia and neutropenia (19, 26). Therefore, we investigated the drug interactions between CI-994 and conventional anticancer drugs (etoposide, cisplatin and 4-HC) that are commonly used for the treatment of AT/RT patients (23). We used isobologram analysis to determine which anticancer drugs have synergistic effects when combined with CI-994. Among the three combinations, the combination treatment of CI-994 with etoposide exerted the strongest effect in a dose-dependent manner. Despite the molecular heterogeneity of AT/RT tumors, isobologram analysis of the combination treatment of CI-994 with etoposide showed consistent responses in all AT/RT cells. On the other hand, the combination of CI-994 with cisplatin or 4-HC revealed antagonism in at least one combination ratio in AT/RT cells, and the response of each cell was inconsistent. For these reasons, we conducted this study focusing on the combination treatment of CI-994 with etoposide.
Next, we determined whether CI-994 efficiently inhibits Class I HDAC gene expression and enzyme activities in AT/RT cells. The inhibitory effect of the combination treatment of CI-994 with etoposide was relatively similar to the effect of CI-994 alone. As a result, there might be other regulatory factors that could cause the synergistic effect of the combination treatment. We speculated that two target molecules modify each other’s activity, thus generating the observed synergy. Previous studies have suggested that acetylation seems to influence the efficacy of etoposide, as both HDAC and histone acetyltransferase (HAT) activities increase the efficacy of etoposide (33). There is further evidence that HDAC1/2 complexes with Topo II modify each other’s activity in vitro and in vivo (34). Topo II-associated HDAC activity was reduced by a specific HDAC inhibitor, and the combination treatment could increase cytotoxicity (34). Etoposide induces apoptosis by inhibiting the Topo II cleavage complexes, leading to the accumulation of DNA damage (33). In addition, CI-994 and other HDACi have shown to induce apoptosis through post-induction suppression of NF-κB or C-Myc-mediated transcription (35, 36). Taken together, we assumed that the synergistic interaction between CI-994 and etoposide is mediated through Topo II (37). Our results showed considerably decreased Topo II expression and increased Ac-H3 expression in response to the combination treatment, suggesting the possibility that apoptosis may be elevated by these signaling pathways. C-Myc may be involved in this signal pathways, not by NF-κB related signal pathway.
In summary, our results demonstrate that the combination treatment of CI-994 with etoposide exerts a synergistic anticancer effect by promoting apoptosis of AT/RT cells through the modulation of Topo II and Ac-H3 in vitro. Although the use of more selective HDAC1 inhibitors and the addition of animal experiments remain challenges, our results support the possibility that the combination treatment of CI-994 with etoposide deserves further attention as a therapeutic option for pediatric AT/RT.
Data Availability Statement
The original contributions presented in the study are included in the article/Supplementary Material. Further inquiries can be directed to the corresponding author.
Ethics Statement
The studies involving human samples were reviewed and approved by the Institutional Review Board (IRB) of the Seoul National University Hospital (SNUH IRB approval No. 1707-095-878). The patients/participants provided their written informed consent to participate in this study.
Author Contributions
S-KK supervised this study. SC designed the experiment. HK performed the experiment. SC and EK analyzed the data. SC and HK wrote the manuscript. JP, K-HK, SC and JL reviewed and edited the draft. All authors contributed to the article and approved the submitted version.
Funding
This work was supported by the National Research Foundation of Korea (NRF) grant funded by the Korean government (MSIT) (2019R1F1A1063068) and by grants from the National Cancer Center, Republic of Korea (NCC-1810861-1).
Conflict of Interest
The authors declare that the research was conducted in the absence of any commercial or financial relationships that could be construed as a potential conflict of interest.
Acknowledgments
We are grateful to Sung-hwan Bae for graphic illustration.
Supplementary Material
The Supplementary Material for this article can be found online at: https://www.frontiersin.org/articles/10.3389/fonc.2021.648023/full#supplementary-material
References
1. Louis DN, Perry A, Reifenberger G, von Deimling A, Figarella-Branger D, Cavenee WK, et al. The 2016 World Health Organization Classification of Tumors of the Central Nervous System: A Summary. Acta Neuropathol (2016) 131(6):803–20. doi: 10.1007/s00401-016-1545-1
2. Biswas A, Kashyap L, Kakkar A, Sarkar C, Julka PK. Atypical Teratoid/Rhabdoid Tumors: Challenges and Search for Solutions. Cancer Manag Res (2016) 8:115–25. doi: 10.2147/CMAR.S83472
3. Richardson EA, Ho B, Huang A. Atypical Teratoid Rhabdoid Tumour: From Tumours to Therapies. J Korean Neurosurg Soc (2018) 61(3):302–11. doi: 10.3340/jkns.2018.0061
4. Versteege I, Sevenet N, Lange J, Rousseau-Merck MF, Ambros P, Handgretinger R, et al. Truncating Mutations of hSNF5/INI1 in Aggressive Paediatric Cancer. Nature (1998) 394(6689):203–6. doi: 10.1038/28212
5. Torchia J, Golbourn B, Feng S, Ho KC, Sin-Chan P, Vasiljevic A, et al. Integrated (Epi)-Genomic Analyses Identify Subgroup-Specific Therapeutic Targets in CNS Rhabdoid Tumors. Cancer Cell (2016) 30(6):891–908. doi: 10.1016/j.ccell.2016.11.003
6. Johann PD, Erkek S, Zapatka M, Kerl K, Buchhalter I, Hovestadt V, et al. Atypical Teratoid/Rhabdoid Tumors Are Comprised of Three Epigenetic Subgroups With Distinct Enhancer Landscapes. Cancer Cell (2016) 29(3):379–93. doi: 10.1016/j.ccell.2016.02.001
7. Knipstein JA, Birks DK, Donson AM, Alimova I, Foreman NK, Vibhakar R. Histone Deacetylase Inhibition Decreases Proliferation and Potentiates the Effect of Ionizing Radiation in Atypical Teratoid/Rhabdoid Tumor Cells. Neuro Oncol (2012) 14(2):175–83. doi: 10.1093/neuonc/nor208
8. Eyupoglu IY, Savaskan NE. Epigenetics in Brain Tumors: HDACs Take Center Stage. Curr Neuropharmacol (2016) 14(1):48–54. doi: 10.2174/1570159X13666151030162457
9. Dembla V, Groisberg R, Hess K, Fu S, Wheler J, Hong DS, et al. Outcomes of Patients With Sarcoma Enrolled in Clinical Trials of Pazopanib Combined With Histone Deacetylase, Mtor, Her2, or MEK Inhibitors. Sci Rep (2017) 7(1):15963. doi: 10.1038/s41598-017-13114-8
10. Eckschlager T, Plch J, Stiborova M, Hrabeta J. Histone Deacetylase Inhibitors as Anticancer Drugs. Int J Mol Sci (2017) 18(7):1414. doi: 10.3390/ijms18071414
11. Suraweera A, O’Byrne KJ, Richard DJ. Combination Therapy With Histone Deacetylase Inhibitors (Hdaci) for the Treatment of Cancer: Achieving the Full Therapeutic Potential of Hdaci. Front Oncol (2018) 8:92. doi: 10.3389/fonc.2018.00092
12. Duan H, Zhou K, Zhang Y, Yue P, Wang T, Li Y, et al. HDAC1 was Involved in Placental Breast Cancer Resistance Protein Regulation In Vitro: A Preliminary Study. J Cell Mol Med (2019) 23(8):5818–21. doi: 10.1111/jcmm.14414
13. Sredni ST, Halpern AL, Hamm CA, Bonaldo Mde F, Tomita T. Histone Deacetylases Expression in Atypical Teratoid Rhabdoid Tumors. Childs Nerv Syst (2013) 29(1):5–9. doi: 10.1007/s00381-012-1965-8
14. Perla A, Fratini L, Cardoso PS, Nor C, Brunetto AT, Brunetto AL, et al. Histone Deacetylase Inhibitors in Pediatric Brain Cancers: Biological Activities and Therapeutic Potential. Front Cell Dev Biol (2020) 8:546. doi: 10.3389/fcell.2020.00546
15. Kerl K, Ries D, Unland R, Borchert C, Moreno N, Hasselblatt M, et al. The Histone Deacetylase Inhibitor SAHA Acts in Synergism With Fenretinide and Doxorubicin to Control Growth of Rhabdoid Tumor Cells. BMC Cancer (2013) 13:286. doi: 10.1186/1471-2407-13-286
16. Thomas M, Clarhaut J, Tranoy-Opalinski I, Gesson JP, Roche J, Papot S. Synthesis and Biological Evaluation of Glucuronide Prodrugs of the Histone Deacetylase Inhibitor CI-994 for Application in Selective Cancer Chemotherapy. Bioorg Med Chem (2008) 16(17):8109–16. doi: 10.1016/j.bmc.2008.07.048
17. Loprevite M, Tiseo M, Grossi F, Scolaro T, Semino C, Pandolfi A, et al. In Vitro Study of CI-994, a Histone Deacetylase Inhibitor, in non-Small Cell Lung Cancer Cell Lines. Oncol Res (2005) 15(1):39–48. doi: 10.3727/096504005775082066
18. Thurn KT, Thomas S, Moore A, Munster PN. Rational Therapeutic Combinations With Histone Deacetylase Inhibitors for the Treatment of Cancer. Future Oncol (2011) 7(2):263–83. doi: 10.2217/fon.11.2
19. Pauer LR, Olivares J, Cunningham C, Williams A, Grove W, Kraker A, et al. Phase I Study of Oral CI-994 in Combination With Carboplatin and Paclitaxel in the Treatment of Patients With Advanced Solid Tumors. Cancer Invest (2004) 22(6):886–96. doi: 10.1081/CNV-200039852
20. Lee C, Lee J, Choi SA, Kim SK, Wang KC, Park SH, et al. M1 Macrophage Recruitment Correlates With Worse Outcome in SHH Medulloblastomas. BMC Cancer (2018) 18(1):535. doi: 10.1186/s12885-018-4457-8
21. Choi SA, Kim SK, Lee JY, Wang KC, Lee C, Phi JH. LIN28B Is Highly Expressed in Atypical Teratoid/Rhabdoid Tumor (at/RT) and Suppressed Through the Restoration of SMARCB1. Cancer Cell Int (2016) 16:32. doi: 10.1186/s12935-016-0307-4
22. Chou TC. Drug Combination Studies and Their Synergy Quantification Using the Chou-Talalay Method. Cancer Res (2010) 70(2):440–6. doi: 10.1158/0008-5472.CAN-09-1947
23. Jangra A, Choi SA, Yang J, Koh EJ, Phi JH, Lee JY, et al. Disulfiram Potentiates the Anticancer Effect of Cisplatin in Atypical Teratoid/Rhabdoid Tumors (at/RT). Cancer Lett (2020) 486:38–45. doi: 10.1016/j.canlet.2020.05.006
24. Li Y, Seto E. Hdacs and HDAC Inhibitors in Cancer Development and Therapy. Cold Spring Harb Perspect Med (2016) 6(10):48:38–45. doi: 10.1101/cshperspect.a026831
25. LoRusso PM, Demchik L, Foster B, Knight J, Bissery MC, Polin LM, et al. Preclinical Antitumor Activity of CI-994. Invest New Drugs (1996) 14(4):349–56. doi: 10.1007/BF00180810
26. Graziano MJ, Pilcher GD, Walsh KM, Kasali OB, Radulovic L. Preclinical Toxicity of a New Oral Anticancer Drug, CI-994 (Acetyldinaline), in Rats and Dogs. Invest New Drugs (1997) 15(4):295–310. doi: 10.1023/A:1005937502511
27. Livyatan I, Meshorer E. The HDAC Interaction Network. Mol Syst Biol (2013) 9:671. doi: 10.1038/msb.2013.33
28. Ho B, Johann PD, Grabovska Y, De Dieu Andrianteranagna MJ, Yao F, Fruhwald M, et al. Molecular Subgrouping of Atypical Teratoid/Rhabdoid Tumors-A Reinvestigation and Current Consensus. Neuro Oncol (2020) 22(5):613–24. doi: 10.1093/neuonc/noz235
29. Seo YJ, Kang Y, Muench L, Reid A, Caesar S, Jean L, et al. Image-Guided Synthesis Reveals Potent Blood-Brain Barrier Permeable Histone Deacetylase Inhibitors. ACS Chem Neurosci (2014) 5(7):588–96. doi: 10.1021/cn500021p
30. Choi SA, Lee C, Kwak PA, Park CK, Wang KC, Phi JH, et al. Histone Deacetylase Inhibitor Panobinostat Potentiates the Anti-Cancer Effects of Mesenchymal Stem Cell-Based sTRAIL Gene Therapy Against Malignant Glioma. Cancer Lett (2019) 442:161–9. doi: 10.1016/j.canlet.2018.10.012
31. Choi SA, Kwak PA, Kim SK, Park SH, Lee JY, Wang KC, et al. In Vivo Bioluminescence Imaging for Leptomeningeal Dissemination of Medulloblastoma in Mouse Models. BMC Cancer (2016) 16(1):723. doi: 10.1186/s12885-016-2742-y
32. Yang SY, Choi SA, Lee JY, Park AK, Wang KC, Phi JH, et al. miR-192 Suppresses Leptomeningeal Dissemination of Medulloblastoma by Modulating Cell Proliferation and Anchoring Through the Regulation of DHFR, Integrins, and CD47. Oncotarget (2015) 6(41):43712–30. doi: 10.18632/oncotarget.6227
33. Montecucco A, Zanetta F, Biamonti G. Molecular Mechanisms of Etoposide. EXCLI J (2015) 14:95–108. doi: 10.18632/oncotarget.6227
34. Tsai SC, Valkov N, Yang WM, Gump J, Sullivan D, Seto E. Histone Deacetylase Interacts Directly With DNA Topoisomerase II. Nat Genet (2000) 26(3):349–53. doi: 10.1038/81671
35. Guo D, Hong D, Wang P, Wang J, Chen L, Zhao W, et al. Histone Deacetylase Inhibitor CI-994 Inhibits Osteoclastogenesis Via Suppressing NF-kappaB and the Downstream c-Fos/NFATc1 Signaling Pathways. Eur J Pharmacol (2019) 848:96–104. doi: 10.1016/j.ejphar.2019.01.021
36. Nebbioso A, Carafa V, Conte M, Tambaro FP, Abbondanza C, Martens J, et al. C-Myc Modulation and Acetylation Is a Key Hdac Inhibitor Target in Cancer. Clin Cancer Res (2017) 23(10):2542–55. doi: 10.1158/1078-0432.CCR-15-2388
Keywords: atypical teratoid/rhabdoid tumor, combination treatment, HDAC1 inhibition, synergism, topoisomerase II
Citation: Kim HY, Choi SA, Koh EJ, Kim KH, Phi JH, Lee JY and Kim S-K (2021) Combination Treatment of CI-994 With Etoposide Potentiates Anticancer Effects Through a Topoisomerase II-Dependent Mechanism in Atypical Teratoid/Rhabdoid Tumor (AT/RT). Front. Oncol. 11:648023. doi: 10.3389/fonc.2021.648023
Received: 31 December 2020; Accepted: 21 June 2021;
Published: 21 July 2021.
Edited by:
David Nathanson, UCLA David Geffen School of Medicine, United StatesReviewed by:
Carsten Friedrich, Klinikum Oldenburg AöR, GermanyJeffrey Rubens, Johns Hopkins University, United States
Copyright © 2021 Kim, Choi, Koh, Kim, Phi, Lee and Kim. This is an open-access article distributed under the terms of the Creative Commons Attribution License (CC BY). The use, distribution or reproduction in other forums is permitted, provided the original author(s) and the copyright owner(s) are credited and that the original publication in this journal is cited, in accordance with accepted academic practice. No use, distribution or reproduction is permitted which does not comply with these terms.
*Correspondence: Seung-Ki Kim, nsthomas@snu.ac.kr
†These authors have contributed equally to this work